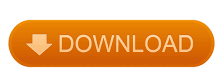

Only a small portion of the microorganisms have been isolated, showing their huge potential as a natural product reservoir whose analysis through innovative techniques has just begun.
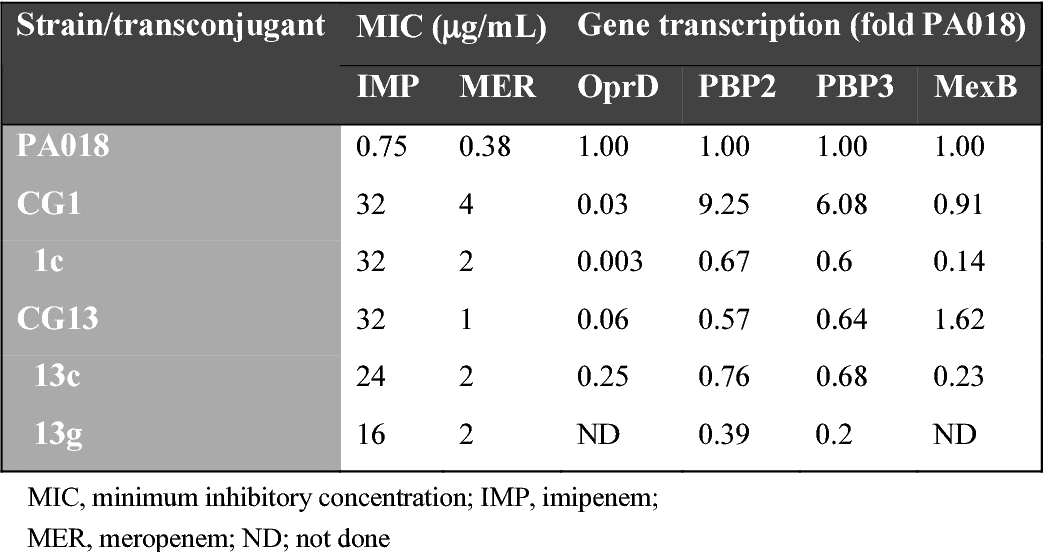
The examples cited here show that targeting PG synthesis by various modes of action is often used by microbes in the environment, and demonstrates once again that natural products are our best source of antibiotics. This mode of action will not be discussed further in this review and we refer the reader to recent reviews on the subject. More recently, a newly discovered natural product, teixobactin, isolated from an uncultured soil microorganism (related to Aquabacteria), was found to inhibit cell wall synthesis by binding to the cell wall precursors lipid II and lipid III. In addition to penicillin, several other antibiotics (glycopetides, lactibiotics, etc.) inhibit the last stage of peptidoglycan polymerization (GT and TP) by binding to the lipid II substrate, the last monomeric precursor of the peptidoglycan polymer, and preventing its use by the polymerases. Resistance affects all antibiotic classes, and the emergence of multidrug resistant bacteria (MDR) has become a major public health crisis worldwide therefore, the battle against MDR strains is a continuous endeavor that should explore all the present possibilities and develop new ones in order to identify new antibiotic classes with low resistance potential. However, the widespread resistance to most β-lactams, particularly that mediated by β-lactamases, limits their efficacy unless they are used in combination with β-lactamase inhibitors, the most recent of which are mostly non-β-lactams. Since the discovery of penicillin and its wide utilization in medicine in the 1940s, several new classes and generations of related β-lactam antibiotics have been developed to successfully fight the emerging resistant strains. Most of the steps in the PG biosynthesis pathway (from uridine diphosphate (UDP)-GlcNAc to the polymeric sacculus) are essential and some of them have been exploited as antibacterial targets, but the most successful of all and the most extensively targeted are the PBPs, named after the “miraculous” penicillin antibiotic that inactivates these enzymes. Schematic representation of the glycosyltransferase and transpeptidase reactions catalyzed by penicillin-binding proteins. In this paper, we present a general background on the GTs and TPs/PBPs, a survey of recent issues of bacterial resistance and a review of recent works describing new inhibitors of these enzymes. Developing screening strategies to take advantage of these mechanisms could lead to new inhibitors of PG assembly.
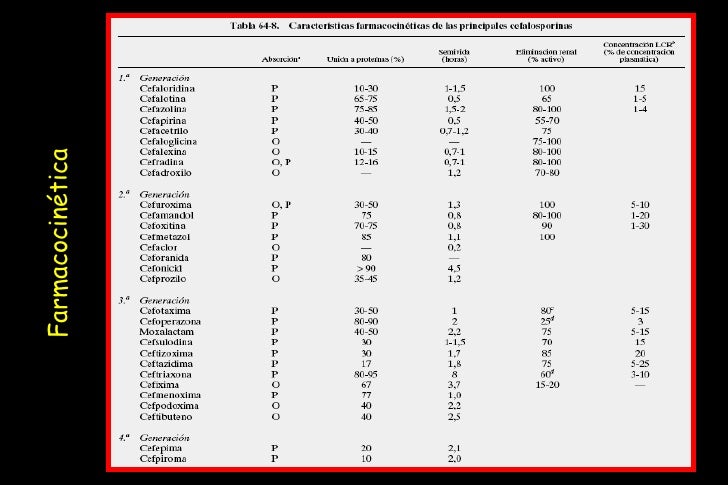
Perturbation of these systems could lead to lethal effects. The overall evidence shows that PBPs are part of multiprotein machineries whose activities are modulated by cofactors. In addition to traditional β-lactams, other classes of molecules (non-β-lactams) that inhibit PBPs are now emerging, opening new perspectives for tackling the resistance problem while taking advantage of these valuable targets, for which a wealth of structural and functional knowledge has been accumulated. In contrast, the TP/PBP module has been successfully targeted by β-lactam derivatives, but the extensive use of these antibiotics has selected resistant bacterial strains that employ a wide variety of mechanisms to escape the lethal action of these antibiotics. Although good progress has been made in terms of the functional and structural understanding of GT, finding a clinically useful antibiotic against them has been challenging until now. Both activities are essential for the synthesis of a functional stress-bearing PG shell. It is assembled by the glycosyltransferase (GT) and transpeptidase (TP) activities of multimodular penicillin-binding proteins (PBPs) within multiprotein complex machineries. Peptidoglycan (PG) is an essential macromolecular sacculus surrounding most bacteria.
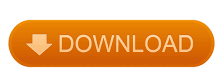